- Ventilation
- Posted
Thin air
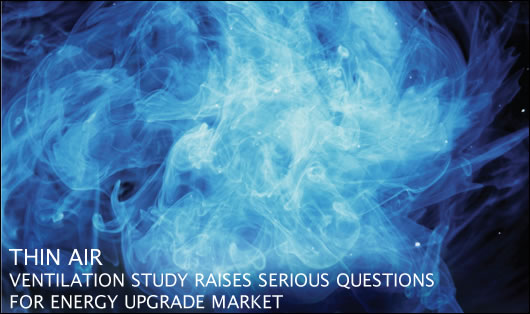
Independent integrated sustainable design consultant Xavier Dubuisson explains why heed must be paid to a recent study on the threats that misjudged energy upgrade work can pose to indoor air quality.Whilst the Home Energy Saving scheme and other energy retrofitting initiatives are essential in addressing the inefficiency of our existing building stock, we are in danger of missing a key element in making our homes more comfortable, healthier and more sustainable places to live in. Tens of thousands of houses undergoing insulation upgrades are likely to become significantly more airtight, and with no encouragement to address ventilation, the potential is there for a reduction in indoor air quality (IAQ). Most people are very sensitive to thermal comfort and many householders have realised that installing insulation and reducing draughts will make their homes cosier and will benefit their pocket. Poor air quality seems to be a nuisance that people are less sensitive to – unless it leads to bad smells. They may not realise it can be addressed without compromising on thermal comfort or heating bills.
One should also say that the regulatory framework doesn’t really help them make the right choices either, since it limits itself to recommending an incredible amount of holes in the wall or installing a mechanical ventilation system with heat recovery (MVHR) – which may not prove viable in many retrofits. And, in the absence of a grant for efficient ventilation systems, holes in the walls remain the default ventilation option for most. It appears that good indoor air quality and energy performance are objectives which the building sector, the regulators and even SEAI are finding difficult to reconcile. There is a remarkable lack of information about ventilation on the Home Energy Saving scheme web pages or anywhere else on SEAI’s website (try ‘ventilation’ in its search engine). This is not helped by the fact that ventilation is nowhere to be seen as a priority for public R&D, despite the fact that it is a public health issue.
Learning from simulating
In this context, the publication of a report by Aereco on a study carried out by the ventilation company is very useful in advancing our understanding of the impact of retrofitting houses on IAQ. The study uses dynamic simulation to assess the effect of increasing levels of airtightness and different ventilation systems on air quality and energy efficiency, taking a 70m2 mid-terrace house built in the 1950s in Dublin as a case study. This house was part of a sample of over 30 Irish houses that were the subject of the European research project on ventilation, Avash (Advanced Ventilation Approaches for Social Housing). The simulation was carried out by Aereco’s R&D department using Siren, a software package developed by the Centre Scientifique et Technique du Bâtiment (CSTB), a French state-owned body that delivers technical approvals for building technology. Siren simulates different scenarios of dwelling configuration, occupancy and human activity, weather conditions, air infiltration and ventilation systems, to measure their impact on air quality and thermal losses. The output of the simulation include cumulated CO2 exposure, levels of volatile organic compounds (VOCs) in each room, air flow rates in each room, evolution of indoor relative humidity, and a measure of the ventilation heat losses. Siren is highly regarded by the scientific community and industry, and has been demonstrated to provide results very close to reality in the framework of monitoring projects, with less than 10% discrepancy.
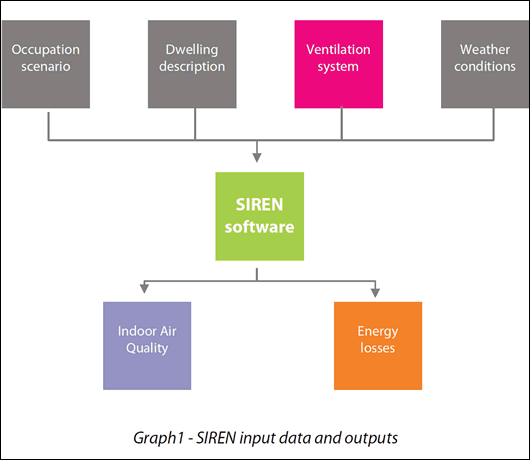
The case study house is two-storey, it has a floor area of 69m2 and a volume of 170m3, and is occupied by a family of four. A standard scenario of CO2, humidity and VOC releases were established on the basis of typical occupancy and activity patterns for this type of house and family. Four levels of airtightness were considered (cases A, B, C and D with airtightness levels at 10, 7.8, 5 and 3 ACH at 50 Pa respectively), representing different levels of retrofit and fabric type. For each case, the performance of several ventilation methods were analysed, selecting from the following options:
• wall vents in dry rooms and kitchen with a 100mm diameter hole;
• as above, but with wall vents blocked by occupants to avoid draughts;
• wall vents in dry rooms and intermittent fans in wet rooms (no passive vents were considered in wet rooms);
• constant air flow mechanical extract ventilation (MEV), using existing passive vents in dry rooms and centralised mechanical extraction from wet rooms
• Demand-controlled MEV with humidity-sensitive air inlets in dry rooms and humidity-sensitive extract grilles in wet rooms (DC MEV);
• Mechanical ventilation with heat recovery with a 90% theoretical heat recovery ratio, to which an in-use efficiency factor of 0.85 is applied to reflect heat losses in the ductwork (according to the UK’s SAP manual 2009, table 4h).
The results of the dynamic simulation of different ventilation methods for each case of airtightness are discussed hereafter in terms of IAQ (relative humidity, CO2, VOCs) and ventilation heat losses. While some of the approaches to ventilation selected do not fully comply with Part F (2009) of the Building Regulations, they were selected as reflecting probable choices by occupants/owners in a retrofit situation.
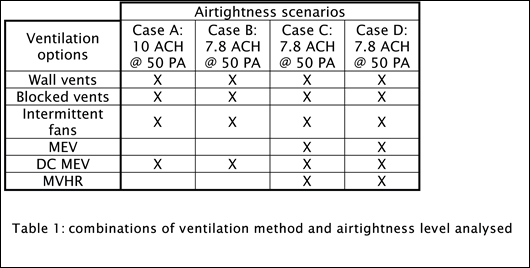
Relative humidity and moisture
The dynamic simulation for each combination above computes two useful indicators of IAQ associated to humidity:
• total number of hours during which relative humidity (RH) is above 75% in wet rooms (kitchen and bathroom) during the heating season;
• hourly rates of relative humidity in wet rooms, converted to moving averages which according to the UK Part F 2010 criteria should never exceed 85% on any given day, 75% on any five day period, and 65% on any 30-day period.
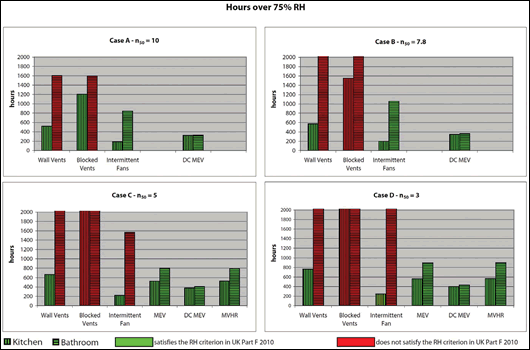
The following observations can be made from the simulation results:
• The UK Part F 2010 criteria are not complied with in bathrooms without mechanical extracts. RH is acceptable in kitchens with wall vents or where the level of air leakage is high, such as in case A.
• In cases B, C & D, persistently high levels of RH are maintained in kitchens and bathrooms where the wall vents have been blocked. Given that surface temperatures below 17C will lead to condensation when RH is at 75%, poorly insulated or poorly heated homes with such levels of ventilation are very likely to experience mould growth. As can be expected, the analysis shows that this problem is exacerbated with increased levels of airtightness.
• More alarmingly, the simulation study indicates that the approach relying on intermittent fans only (without background ventilation) is becoming ineffective in the bathroom once the house is reasonably airtight (cases C and D). In case D, RH exceeds 75% in the bathroom for circa 2,400 hours, equivalent to 100 days out of the 233-day heating season. This puts a strong interrogation point on the very common practice of bathroom ventilation.
• ‘Always on’ mechanical ventilation systems perform adequately, with DC MEV showing particularly good results since its ability to increase its extraction rate to deal with high levels of RH leads to a swift removal of excess moisture and lower ongoing levels of relative humidity. This is demonstrated in the graph below which shows how DC MEV maintains RH within the comfort zone (below 55%) in the kitchen and bathroom most of the time during the heating season, even in the most airtight house.
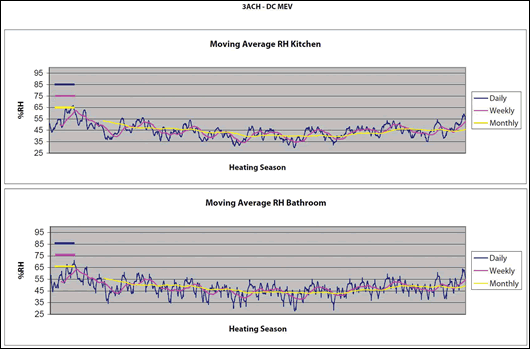
CO2 and volatile organic compounds (VOCs) exposure
Siren compiles levels of exposure to CO2 as the number of hours during which CO2 concentrations are over 2,000 ppm (part per million) – this is considered the threshold for poor air quality – aggregated over the heating season and expressed in ppm.hours. The recommended exposure threshold for that period is 500,000 ppm.hours of CO2, according to the CSTB. Most ventilation methods comply with this recommendation, however the lack of ventilation in the combination of blocked vents and high airtightness leads to CO2 exposure well above the limit. Again this raises the very serious issue of poor IAQ where ventilation is compromised to preserve the thermal comfort of occupants, this being reinforced by increased airtightness post-retrofit.
The science of predicting VOC emission levels is a lot less exact than with CO2 or humidity, and there isn’t a definite consensus on what are acceptable levels of VOC concentration for IAQ purposes. However, the simulation carried out confirms that effective ventilation is essential in reducing levels of exposure and that VOCs pose a health risk in houses with poor ventilation. The simulation results show that DC MEV deals adequately with VOCs, even at low levels of ventilation when RH is low.
Air flows and heat losses
Siren computes the air flows and heat losses associated with air infiltration and intended ventilation for the different combinations of airtightness and ventilation. As these air flows vary all the time – for instance due to wind pressure, air temperature and demand-control – an ‘equivalent air flow’ is computed to allow comparison between different ventilation approaches. This corresponds to a constant airflow resulting in an equivalent amount of ventilation heat losses. The equivalent air flow for MVHR systems also takes into consideration that a certain amount of heat loss is avoided
due to heat recovery. The graphs below show the simulation results.
The first striking result is how important airtightness is to reducing heat losses – halving air leakage reduces ventilation heat losses by approximately 35% (from case A to C). Also, constant mechanical extract ventilation (MEV) offers no heat loss reduction compared to the natural ventilation approaches simulated, including with intermittent fans.
Advanced ventilation strategies clearly show their benefit in terms of thermal efficiency. Demand-controlled MEV consistently outperforms natural ventilation strategies and MEV thanks to its ability to reduce air flows when there is less demand for ventilation. In case C, with good airtightness, the simulations show that DC MEV generates circa 30% less heat loss than in the ‘intermittent fans’ approach while MVHR results in a 47% reduction in heat loss.
Critical lessons
The study contains very important lessons that should be given serious consideration by the regulator, relevant agencies, specifiers and ventilation solution providers. First of all, the results of the study indicate clearly that retrofitting houses poses a real challenge to indoor air quality as the airtightness of refurbished properties increases. The fact that conventional methods of wall vents and intermittent fans appear to fail to adequately deal with moisture levels in wet rooms raises serious concerns. The study also demonstrates that the not uncommon practice of closing or blocking wall vents to preserve thermal comfort has dramatic consequences for air quality in houses. In addition, it demonstrates clearly the benefit of airtightness and efficient mechanical ventilation solutions such as DC MEV and MVHR.
Secondly, this study is a great example of how dynamic simulation using proven tools such as the Siren software can greatly increase our understanding of how effective different ventilation strategies are. In an Irish context, they give us the opportunity to compare the performance of different ventilation systems within similar conditions of climate, occupancy and airtightness at a fraction of the cost of monitoring studies, and almost instantaneously. There is a clear need to learn from studies like this one and tools such as Siren in developing the housing retrofit guidelines when it comes to ventilation, and in reviewing the methodology currently used in Deap to assess the energy performance of various ventilation methods.
For further information email
This email address is being protected from spambots. You need JavaScript enabled to view it. or visit www.aereco.ie to download the full study