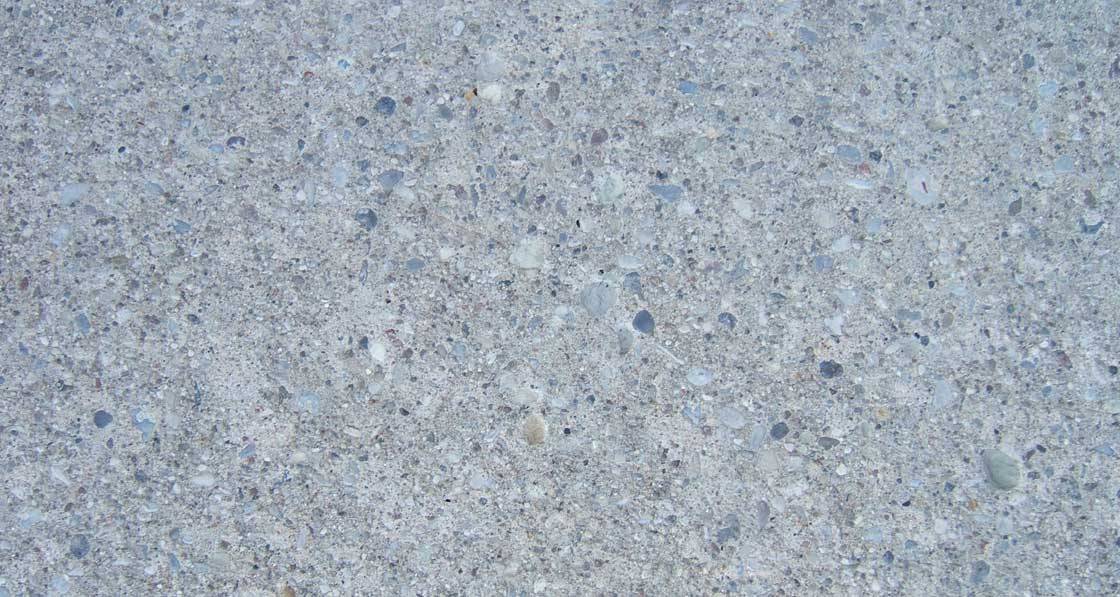
- Guides
- Posted
The PH+ guide to greener concrete - reducing the climate impact of cement and concrete in buildings
Cement is responsible for up to 8% of global carbon emissions, and in this guide, sustainable design expert Jay Stuart looks at ways to minimise its environmental impact through good design, and at some of the alternative, lower carbon cement and concrete products on the market.
This article was originally published in issue 33 of Passive House Plus magazine. Want immediate access to all back issues and exclusive extra content? Click here to subscribe for as little as €10, or click here to receive the next issue free of charge
The operational energy use of new buildings has now been significantly reduced with nearly zero energy building (NZEB) standards, at least in EU countries, and the increasing use of renewable energy. Together these two strategies have reduced the carbon emissions generated in the operation of new buildings. Building regulations are also likely to require zero energy buildings (ZEB) in the near future, which should reduce the carbon emissions from operational energy use to zero in new buildings.
For several years attention has been shifting to the embodied carbon of the materials, components and systems used in the construction of buildings. Research shows that the embodied carbon of the construction stage is between 30% to 70% of total carbon emissions during the life of a building. Embodied carbon is therefore the next logical focus for the building regulations.
Some planning authorities in the UK and Europe are already requesting calculations of embodied carbon at planning stage.
Embodied carbon evaluation may become a differentiator in the planning process before it is written into building regulations. Most building rating systems such as BREEAM, LEED, Green Star, and the Irish Green Building Council’s Home Performance Index recognise embodied carbon measurement and mitigation as part of minimising a building’s life cycle impacts.
As we build increasingly energy efficient buildings that use less operating energy and more renewable energy, the embodied carbon of the construction stage becomes a higher proportion of the building’s total life cycle carbon emissions. If the materials used actively sequester carbon dioxide, then we can also use buildings to remove CO2 from the atmosphere.
Cemfree low carbon concrete was used by the Environment Agency to achieve an 88% embodied carbon reduction in sea defences at Foulton Hall, Essex.
Lower carbon cement & concrete
To achieve climate change targets, it is imperative that we address embodied carbon. The use of life cycle analysis (LCA) tools to assess the carbon emissions of the whole life of a building is a necessary first step to identify where reductions can be achieved. The detailed information for LCAs will come from research databases such as Ecoinvent (www. ecoinvent.org), which describes itself as “the world’s most consistent and transparent life cycle inventory database”, and from manufacturers’ Environmental Performance Declarations (EPD), which are available for an increasing number of construction products.
Concrete is one the most carbon intensive materials used in construction. This is because large amounts of CO2 are emitted when cement, one of the main ingredients in concrete (along with aggregate and water), is produced by heating limestone (calcium carbonate) to a very high temperature. Cement accounts for about 8% of global carbon emissions, which is about three to four times more than the carbon emissions of global air travel. The embodied carbon of new construction can be reduced with low carbon design mixes for concrete, more efficient designs which use less concrete and the use of other materials and technologies in place of concrete.
The cement and concrete industry has been developing lower carbon cement and concrete for a long time. By using recycled materials, developing design mixes for specific uses, and burning alternative fuels instead of fossil fuels in some cement plants, the industry has reduced the embodied carbon of concrete. Concrete mixes can now be specified which range from about 105 kg to 435 kg of CO2 per cubic metre, depending on the source of the raw materials, cement manufacturing methods and the design mix.
The concrete in the substructure, foundations and superstructure account for about 55% of the embodied carbon in a typical building. The 2012 London Olympics Delivery Authority and contractors worked together to reduce the embodied carbon in all the concrete they used by 24% through careful specification of the design mixes and a further 16% re duction through rationalised design efficiencies of the structures and buildings.
The use of by-products from other industrial processes as substitutes for cement and aggregates is well established, but they are not necessarily what your local Readymix supplier will deliver unless clients, professionals and contractors specify low carbon concrete.
GGBS (ground granulated blast-furnace slag, a byproduct of iron and steel manufacturing) and PFA (pulverised fuel ash, a byproduct of coal power plants) have been used as partial substitutes for ordinary Portland cement (OPC) for decades now, as has construction and demolition waste as a substitute for stone aggregates. These are often specified because they cost less than OPC or newly quarried rock. They also happen to have lower embodied carbon than OPC or newly quarried rock. In any project early discussions on this issue with structural engineers, contractors and concrete suppliers will enable the use of appropriate local design mix solutions with much less embodied carbon.
Passive House Plus has previously reported about concrete block manufacturers switching all of their production to 50% GGBS without increasing costs, adversely affecting quality or slowing down production time, thanks to an accelerant developed by GGBS manufacturer Ecocem. A number of insulating concrete formwork suppliers are also offering 50+% GGBS in their formwork, while some precast concrete manufacturers are using up to 70% GGBS with 30% OPC in their products, citing increased resistance to chemical and chloride attack, improved long term strength gain and lower heat of hydration enabling large volume pours due to reduced risk of cracking. Other than embodied CO2 savings, GGBS has often been used in concrete because of the lower cost, increased strength, longer life, smoother finish and white colour it gives to concrete.
Aided by the development of new accelerants, the controlled conditions of precasting and blockmaking allow a higher proportion of GGBS, but the designer/specifier must know what is possible and specify it correctly. Technically, it is possible to substitute 100% of OPC with GGBS, though in the past this has been impractical due to drying times which exponentially increase once the share rises above 30 to 50%, depending on the application. However, the development of new accelerant additives is causing this to rapidly change, and products which entirely substitute OPC with 95% GGBS and a 5% alkali reactor, such as Cemfree from DB Group (Holdings) Ltd, are now commercially available in the UK.
Research and development continues and there are new processes and products which can further reduce the impact of concrete. O.C.O Technology Ltd in the UK have recently perfected a process which produces M-LS (manufactured limestone), a “carbon negative” aggregate that can be used in concrete to replace stone aggregates. It uses CO2 gas to treat thermal wastes (pollution control wastes from industrial smokestacks) and convert them into stable calcium carbonates which are blended with binders and fillers to form M-LS. This product can significantly reduce the impact of concrete when it is specified and used in place of stone aggregates. The process permanently captures and sequesters significant amounts of CO2 and, if combined with a high GGBS cement mix, a much lower carbon concrete can be produced.
O.C.O have three plants in the UK and three active development projects in other countries. But like everything in the cement and concrete industry, if it’s not made locally the carbon emissions of transporting anything this heavy any distance by road may outweigh the lower embodied carbon of the materials.
You may have seen various articles and news items stating that a ‘bio-concrete’ or ‘bio-masonry’ has been developed which uses bacteria to make a new type of low carbon concrete. This could be a wonderful solution, but the research is at an early stage and the best results are difficult to replicate. To the best of my knowledge it is not commercially available as a product or process. It may yet prove to be useful but at the moment it is in the R&D phase. It may be ‘bio’, but we don’t yet have evidence to indicate it is an ecologically better product. I was a partner in an EU funded research project called ‘Eco-Cement’ (2012-2015), and the soil bacteria that was the focus of this research used urea to produce enzymes which form carbonate crystals which bind particles to form something akin to concrete.
In our trials we made a mortar, a plaster and a tile, but they were not very strong. Our role in the project was to do the LCA assessment of our process to assess its embodied carbon and how successful our process was in producing an ‘eco-cement’. We completed an early assessment and discovered that the only available source of urea was made from fossil fuel gas. This completely blew the product up into a high embodied carbon material, worse than ordinary Portland cement.
We did solve this urea problem by creating our own ‘bio-urea’ in a complex integrated industrial process to make an ‘eco-cement’, but this was a completely theoretical exercise and no such plant exists. Much work remains to be done to increase its strength and durability as well as lower its embodied carbon.
Since this ‘eco-cement’ is not available, and if a project is not near a source of GGBS, M-LS or recycled construction and demolition waste, then the designer can also consider minimising or avoiding the use of concrete.
Concrete blocks manufactured using ‘carbon negative’ aggregate from O.C.O Technology Ltd.
Design efficiencies
Thornton Tomasetti, a global engineering consultancy, have recently launched a Revit BIM plug-in for building structures called Beacon which calculates the embodied carbon of concrete in a building’s structural design to inform the design process. If reducing embodied carbon in a building is a design objective, then it is possible to develop more efficient designs which use less concrete and assess their relative embodied carbon.
Accompanying such tools are integrated software systems for the design optimization and dematerialisation of structures, which can lead to more efficient and lower carbon structural design solutions.
Building methods
There are many building methods, technologies and systems which can contribute to the reduction of embodied carbon and they deserve separate articles. I list some of them here and it would be interesting if readers were to send PH+ magazine (info@passivehouseplus. ie) technologies or products they know about which could be added to this list for a future PH+ guide or article.
I will start this list with a few that I know about: vibro-compacted stone foundation systems; floating/raft foundation slabs; metal helical screw or auger piles; CLT timber structures; hempcrete blocks; to name a few.
Foundations
I have included one build method though, as it does represent a greener use of concrete, rather than an alternative to concrete: an insulated slab on vibro-compacted stone. Vibro-compacted stone foundation technology is well developed and has many advantages. There are different techniques but in essence a specialised machine vibrates a pile of stones into the soil until the compacted stones achieve the required bearing capacity.
Low rise buildings can spread the foundation load with a reinforced ‘floating slab’ on a 200 mm layer of compacted stones as was done at the Silken Park housing scheme in west Dublin. Here a thin 100 mm reinforced concrete slab was poured into a ‘passive insulated formwork’ of prefabricated EPS insulation to help achieve the passive house certification. By avoiding conventional trench strip foundations there were cost savings in excavation, carting away subsoil and pouring concrete to fill up the trenches. If the concrete slab also had a high GGBS mix with recycled aggregate or M-LS it would be an extremely low embodied carbon solution.
One alternative to mention in this article, which, in uncrushed form, is a constituent ingredient in concrete: stone. Stone was used structurally in buildings for centuries but it is now mostly used as a thin cladding material. Amir Taha, a UK architect, has recently completed a 6 storey structural stone building in Clerkenwell, London which uses limestone cut at the quarry by stone masons to create columns and beams and other elements of the building. It is estimated that the embodied carbon of this quarried stone is only 15% of the equivalent structure in reinforced concrete.
Stone can last for centuries but reinforced concrete is variously estimated to last 75 to 120 years depending on the design mix.
Galway-based concrete product supplier Coshla Quarries manufacture blockwork as standard featuring 50% GGBS from Ecocem.
Conclusion
It is clear that the need to reduce the environmental impact of embodied carbon in construction is becoming an increasingly significant issue for the industry. To achieve lower embodied carbon construction the industry needs to adopt the specification of low carbon construction methods, materials and processes as an integral part of the briefing, design, specification and procurement stages of a project.
There are many alternatives to concrete which, along with modern methods of concrete production, means there is lots of potential to reduce embodied carbon. But concrete is manufactured locally so the embodied carbon of concrete varies considerably by location, and it needs to be carefully specified to achieve its potential.
The business case for lower embodied carbon buildings is being driven first by environmental assessment and endorsement systems like BREEAM and LEED, followed by the corporate social responsibility and environmental policies of some client organisations which are now increasingly being required by financial institutions. Some planning authorities are asking for embodied carbon information and estimates, and there have been discussions at industry conferences about regulating embodied carbon using the building regulations.
The London Olympics Delivery Authority achieved significant reductions in embodied carbon in its concrete and saved money in the process. Anglian Water has demonstrated capital cost savings of between 30-50% by tracking embodied carbon in their infrastructure projects, which are predominantly constructed in concrete.
It is my opinion and experience that there are more sustainable solutions for every process and product in the building industry, and if used in an integrated design, there can also be significant cost savings. I suggest that reducing the embodied carbon of buildings will result in reduced capital costs of construction when the industry scales up to meet this challenge.
(left to right) The foundation system used at Silken Park consisted of vibro compacted stone; a KORE passive slab system; and consequently a significantly reduced concrete slab pour.
EMBODIED CARBON FOUNDATION COMPARISON
Michael McCarthy of leading cost consultants MMC Quantity Surveyors conducted a comparative analysis of embodied carbon emissions of two common foundation systems in low energy buildings: a passive slab foundation system, and a traditional strip foundation, in both cases for a 100 m2 typical semi-detached house.
The passive slab system in this case consisted of the KORE passive slab of EPS 300 with a ring beam, with 50% GGBS concrete assumed, compared to an insulated strip foundation system made with OPC.
With 50% of cement in the concrete substituted for GGBS, the slab’s total concrete mix achieves a 27% reduction in carbon dioxide equivalent (CO2e) over standard concrete (28N).
Due largely to this fact, a reduction in excavated material, and the substantial reduction in the quantity of concrete required, the system achieves a 51% reduction in embodied CO2e emissions – a reduction from over 17 tonnes to 8.3 tonnes.
In addition, according to MMC the passive slab costs about €2,000 less to construct than the standard strip foundation. If a higher percentage of GGBS is used, along with the likes of OCO’s M-LS aggregate, a substantially higher reduction could be achieved.
That said, it is also possible to achieve significant reductions with strip foundations too, by changing the concrete recipe as per above – albeit without reducing the quantity of concrete, and the additional scope for emissions reductions this offers.